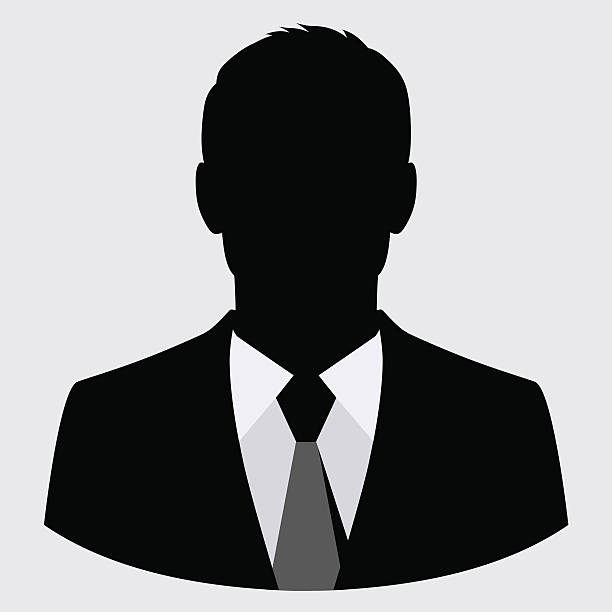
Radhika Nagre,
- Research Scholar Department of Biochemistry, Osmania University, Hyderabad Telangana India
Abstract
Cancer is a prominent cause of mortality globally, and it has the potential to impact any region of the body. The tumor suppressor protein P53 plays a significant role in inhibiting tumor growth by regulating DNA repair, inducing cell cycle arrest, promoting senescence, and triggering apoptosis when confronted with genotoxic stress. P53 is found mutated in 50% of cancers where it loses its tumor suppressive properties and gains oncogenic properties where it functions in a contradictory way in cancer cells when compared to normal cells. The mutated P53 forms oligomers with wtP53 rendering it incapable of carrying out its apoptotic functions and instead uses its prosurvival functions in promoting the tumor growth. Small ligand molecules that can bind the wtP53 and inhibit its oligomerisation with mutant P53 can be a possible therapy for cancer. Another possibility of targeting wtP53 in a cancer cell can be the induction of apoptotic function instead of pro-survival function which can be useful in eliminating the cancer cell whose damage is beyond repair. There are various therapeutic interventions available for cancer therapy but they are not without serious side effects which generates a need to develop drugs that do not have many side effects and that do not harm normal cells. In present times plant based phytochemicals have gained recognition for having many anticancer therapeutic properties among which Morinda citrifolia, also known as noni is found to have many phytochemicals with anticancer effects that can be exploited as potential therapy for cancer. In this study we used two PDBID structures of P53, PDBID-6FF9, containing the N-terminal domain (NTD) and the DNA binding domain (DBD) and PDBID-3SAK containing only the tetramerisation domain (TD) of wtP53 and analysed the docking of these structures with two of the phytochemicals (Damnacanthal, Scopoletin) present in Morinda citrifolia which are known to have potential anticancer therapeutic value. The two ligands were found to interact with the protein and the best docking position with the lowest binding energy was selected and reported in this paper.
Keywords: Morinda citrifolia, Damnacanthal, Scopoletin, Tumor suppressor protein P53, Phytochemicals.
[This article belongs to International Journal of Molecular Biotechnological Research(ijmbr)]

Browse Figures
References
- https://www.who.int/news-room/fact-sheets/detail/cancer
- https://cancer.org/healthy/cancer-causes/genetics/genes-and-cancer/oncogenes-tumor- suppressor-genes.html
- Muhseen, Z. T., & Li, G. (2019). Promising Terpenes as Natural Antagonists of Cancer: An In- Silico Approach. Molecules (Basel, Switzerland), 25(1), 155. https://doi.org/10.3390/molecules25010155
- Kamada, R., Nomura, T., Anderson, C. W., & Sakaguchi, K. (2011). Cancer-associated p53 tetramerization domain mutants: quantitative analysis reveals a low threshold for tumor suppressor inactivation. The Journal of biological chemistry, 286(1), 252–258. https://doi.org/10.1074/jbc.M110.174698
- Liebl, M. C., & Hofmann, T. G. (2021). The Role of p53 Signaling in Colorectal Cancer. Cancers, 13(9), 2125. https://doi.org/10.3390/cancers13092125
- Babamohamadi, M., Babaei, E., Ahmed Salih, B., Babamohammadi, M., Jalal Azeez, H., & Othman, G. (2022). Recent findings on the role of wild-type and mutant p53 in cancer development and therapy. Frontiers in molecular biosciences, 9, 903075. https://doi.org/10.3389/fmolb.2022.903075
- Guo, A., Salomoni, P., Luo, J., Shih, A., Zhong, S., Gu, W., & Pandolfi, P. P. (2000). The function of PML in p53-dependent apoptosis. Nature cell biology, 2(10), 730–736. https://doi.org/10.1038/35036365
- Gomes, A. S., Trovão, F., Andrade Pinheiro, B., Freire, F., Gomes, S., Oliveira, C., Domingues, L., Romão, M. J., Saraiva, L., & Carvalho, A. L. (2018). The Crystal Structure of the R280K Mutant of Human p53 Explains the Loss of DNA Binding. International journal of molecular sciences, 19(4), 1184. https://doi.org/10.3390/ijms19041184
- He, F., Borcherds, W., Song, T., Wei, X., Das, M., Chen, L., Daughdrill, G. W., & Chen, J. (2019). Interaction between p53 N terminus and core domain regulates specific and nonspecific DNA binding. Proceedings of the National Academy of Sciences of the United States of America, 116(18), 8859–8868. https://doi.org/10.1073/pnas.1903077116
- Kim, E., Giese, A., & Deppert, W. (2009). Wild-type p53 in cancer cells: when a guardian turns into a blackguard. Biochemical pharmacology, 77(1), 11–20. https://doi.org/10.1016/j.bcp.2008.08.030
- Tanate, P. (2019). In Silico simulation and analysis of human p53 and non-human translationally controlled tumor protein. ms, Songklanakarin J. Sci. Technol. 41 (1), 229-236.
- Hodos, R. A., Kidd, B. A., Shameer, K., Readhead, B. P., & Dudley, J. T. (2016). Computational approaches to drug repurposing and pharmacology. WIREs Systems Biology and Medicine, 8(3), 186–210. https://doi.org/10.1002/wsbm.1337
- Tong, X., Xu, D., Mishra, R. K., Jones, R. D., Sun, L., Schiltz, G. E., Liao, J., & Yang, G. Y. (2021). Identification of a druggable protein-protein interaction site between mutant p53 and its stabilizing chaperone DNAJA1. The Journal of biological chemistry, 296, 100098. https://doi.org/10.1074/jbc.RA120.014749
- Vousden, K. H., & Prives, C. (2009). Blinded by the Light: The Growing Complexity of p53. Cell, 137(3), 413–431. https://doi.org/10.1016/j.cell.2009.04.037
- Fischer, N. W., Prodeus, A., Malkin, D., & Gariépy, J. (2016). p53 oligomerization status modulates cell fate decisions between growth, arrest and apoptosis. Cell cycle (Georgetown, Tex.), 15(23), 3210–3219. https://doi.org/10.1080/15384101.2016.1241917
- Wang, P., Reed, M., Wang, Y., Mayr, G., Stenger, J. E., Anderson, M. E., Schwedes, J. F., & Tegtmeyer, P. (1994). p53 domains: structure, oligomerization, and transformation. Molecular and cellular biology, 14(8), 5182–5191. https://doi.org/10.1128/mcb.14.8.5182-5191.1994
- Guha, T., & Malkin, D. (2017). Inherited TP53 Mutations and the Li-Fraumeni Syndrome. Cold Spring Harbor perspectives in medicine, 7(4), a026187. https://doi.org/10.1101/cshperspect.a026187
- Tsai, Y. Y., Su, C. H., & Tarn, W. Y. (2021). p53 Activation in Genetic Disorders: Different Routes to the Same Destination. International journal of molecular sciences, 22(17), 9307. https://doi.org/10.3390/ijms22179307
- Wolfrum, P., Fietz, A., Schnichels, S., & Hurst, J. (2022). The function of p53 and its role in Alzheimer’s and Parkinson’s disease compared to age-related macular degeneration. Frontiers in neuroscience, 16, 1029473. https://doi.org/10.3389/fnins.2022.1029473
- Checler, F., & Alves da Costa, C. (2014). p53 in neurodegenerative diseases and brain cancers. Pharmacology & therapeutics, 142(1), 99–113. https://doi.org/10.1016/j.pharmthera.2013.11.009
- Rutherford, J., Chu, C. E., Duddy, P. M., Charlton, R. S., Chumas, P., Taylor, G. R., Lu, X., Barnes, D. M., & Camplejohn, R. S. (2002). Investigations on a clinically and functionally unusual and novel germline p53 mutation. British journal of cancer, 86(10), 1592–1596. https://doi.org/10.1038/sj.bjc.6600269
- Ribeiro, R. C., Sandrini, F., Figueiredo, B., Zambetti, G. P., Michalkiewicz, E., Lafferty, A. R., DeLacerda, L., Rabin, M., Cadwell, C., Sampaio, G., Cat, I., Stratakis, C. A., & Sandrini, R. (2001). An inherited p53 mutation that contributes in a tissue-specific manner to pediatric adrenal cortical carcinoma. Proceedings of the National Academy of Sciences of the United States of America, 98(16), 9330–9335. https://doi.org/10.1073/pnas.161479898
- Stacey, S. N., Sulem, P., Jonasdottir, A., Masson, G., Gudmundsson, J., Gudbjartsson, D. F., Magnusson, O. T., Gudjonsson, S. A., Sigurgeirsson, B., Thorisdottir, K., Ragnarsson, R., Benediktsdottir, K. R., Nexø, B. A., Tjønneland, A., Overvad, K., Rudnai, P., Gurzau, E., Koppova, K., Hemminki, K., Corredera, C., … Stefansson, K. (2011). A germline variant in the TP53 polyadenylation signal confers cancer susceptibility. Nature genetics, 43(11), 1098–1103. https://doi.org/10.1038/ng.926
- Toki, T., Yoshida, K., Wang, R., Nakamura, S., Maekawa, T., Goi, K., Katoh, M. C., Mizuno, S., Sugiyama, F., Kanezaki, R., Uechi, T., Nakajima, Y., Sato, Y., Okuno, Y., Sato-Otsubo, A., Shiozawa, Y., Kataoka, K., Shiraishi, Y., Sanada, M., Chiba, K., … Ito, E. (2018). De Novo Mutations Activating Germline TP53 in an Inherited Bone-Marrow-Failure Syndrome. American journal of human genetics, 103(3), 440–447. https://doi.org/10.1016/j.ajhg.2018.07.020
- Zhang, S., Carlsen, L., Hernandez Borrero, L., Seyhan, A. A., Tian, X., & El-Deiry, W. S. (2022). Advanced Strategies for Therapeutic Targeting of Wild-Type and Mutant p53 in Cancer. Biomolecules, 12(4), 548. https://doi.org/10.3390/biom12040548
- Singh, S., Sharma, B., Kanwar, S. S., & Kumar, A. (2016). Lead Phytochemicals for Anticancer Drug Development. Frontiers in plant science, 7, 1667. https://doi.org/10.3389/fpls.2016.01667
- Chanthira Kumar, H., Lim, X. Y., Mohkiar, F. H., Suhaimi, S. N., Mohammad Shafie, N., & Chin Tan, T. Y. (2022). Efficacy and Safety of Morinda citrifolia L. (Noni) as a Potential Anticancer Agent. Integrative cancer therapies, 21, 15347354221132848. https://doi.org/10.1177/15347354221132848
- Reem, Yusrida, Ibrahim, Arshad, Lim, Laghari. (2017). Morinda citrifolia (noni); a comprehensive review on its industrial uses, pharmacological activities and clinical trials. Arabian Journal of Chemistry, 10(5), ISSN 1878-5352,. https://sciencedirect.com/science/article/pii/S1878535215001902
- The UniProt Consortium (2017). UniProt: the universal protein knowledgebase. Nucleic acids research, 45(D1), D158–D169. https://doi.org/10.1093/nar/gkw1099
- Rose, P. W., Prlić, A., Altunkaya, A., Bi, C., Bradley, A. R., Christie, C. H., Costanzo, L. D., Duarte, J. M., Dutta, S., Feng, Z., Green, R. K., Goodsell, D. S., Hudson, B., Kalro, T., Lowe, R., Peisach, E., Randle, C., Rose, A. S., Shao, C., Tao, Y. P., … Burley, S. K. (2017). The RCSB protein data bank: integrative view of protein, gene and 3D structural information. Nucleic acids research, 45(D1), D271–D281. https://doi.org/10.1093/nar/gkw1000
- Amberger, J. S., & Hamosh, A. (2017). Searching Online Mendelian Inheritance in Man (OMIM): A Knowledgebase of Human Genes and Genetic Phenotypes. Current protocols in bioinformatics, 58, 1.2.1–1.2.12. https://doi.org/10.1002/cpbi.27
- Puthenveetil, R., & Vinogradova, O. (2019). Solution NMR: A powerful tool for structural and functional studies of membrane proteins in reconstituted environments. The Journal of biological chemistry, 294(44), 15914–15931. https://doi.org/10.1074/jbc.REV119.009178
- Doogue, M. P., & Polasek, T. M. (2013). The ABCD of clinical pharmacokinetics. Therapeutic advances in drug safety, 4(1), 5–7. https://doi.org/10.1177/2042098612469335
- Daina, A., Michielin, O. & Zoete, V. SwissADME: a free web tool to evaluate pharmacokinetics, drug-likeness and medicinal chemistry friendliness of small molecules. Sci Rep 7, 42717 (2017). https://doi.org/10.1038/srep42717
- Kim, S., Chen, J., Cheng, T., Gindulyte, A., He, J., He, S., Li, Q., Shoemaker, B. A., Thiessen, P. A., Yu, B., Zaslavsky, L., Zhang, J., & Bolton, E. E. (2021). PubChem in 2021: new data content and improved web interfaces. Nucleic acids research, 49(D1), D1388–D1395. https://doi.org/10.1093/nar/gkaa971
- Gencel-Augusto, J., & Lozano, G. (2020). p53 tetramerization: at the center of the dominant- negative effect of mutant p53. Genes & development, 34(17-18), 1128–1146. https://doi.org/10.1101/gad.340976.120
- Wang, G., & Fersht, A. R. (2015). Propagation of aggregated p53: Cross-reaction and coaggregation vs. seeding. Proceedings of the National Academy of Sciences of the United States of America, 112(8), 2443–2448. https://doi.org/10.1073/pnas.1500262112
- Roemer K. (1999). Mutant p53: gain-of-function oncoproteins and wild-type p53 inactivators. Biological chemistry, 380(7-8), 879–887. https://doi.org/10.1515/BC.1999.108
- Jabir, N. R., Rehman, M. T., Alsolami, K., Shakil, S., Zughaibi, T. A., Alserihi, R. F., Khan, M. S., AlAjmi, M. F., & Tabrez, S. (2021). Concatenation of molecular docking and molecular simulation of BACE-1, γ-secretase targeted ligands: in pursuit of Alzheimer’s treatment. Annals of medicine, 53(1), 2332–2344. https://doi.org/10.1080/07853890.2021.2009124
- Rosignoli, S., & Paiardini, A. (2022). Boosting the Full Potential of PyMOL with Structural Biology Plugins. Biomolecules, 12(12), 1764. https://doi.org/10.3390/biom1212176
Volume | 01 |
Issue | 02 |
Received | August 24, 2023 |
Accepted | September 11, 2023 |
Published | October 20, 2023 |